Key Points
- Fatigue develops temporarily during the most intense periods of a football game, as well as towards the end of a game, and the two types of fatigue are related to different physiological systems.
- Temporary fatigue is proposed to be caused by muscle acidification and depolarization of the muscle resting membrane potential, and fatigue at the end of a game may associate with depleted muscle glycogen stores in individual muscle fibres or muscle cell compartments.
- Aerobic high intensity training and speed endurance training are efficient fatigue resistance training strategies in elite football.
- Intense intermittent training has been shown to improve a wide range of physiological systems directly or indirectly related to both endurance and high intensity exercise performance in trained athletes.
- Large individual variations in game demands and fatigue profiles are present in football, which suggests a greater emphasis on individual fitness training for elite football players.
Introduction
Football match-play is an intermittent multiple-sprint sport with frequent changes in activity where prolonged intermittent exercise is conjoined with short-term periods encompassing high intensity running and explosive football-specific actions (Mohr et al., 2003). Thus, a football game demands a high endurance capacity, as well as ability to repeatedly perform at maximal and near-maximal exercise intensity. This notion is supported by a number of scientific studies reporting a high taxation of both the aerobic and anaerobic energy pathways during a football game (for review see Bangsbo et al., 2006a; Bangsbo, 2014a).
The high physiological loading has also been shown to provoke different types of fatigue throughout the course of a football game (Mohr et al., 2005). For example has it been demonstrated that top-class football players fatigue temporarily during the most intense periods of a game, as well as in the final phase of a game, which is suggested to associate with different physiological mechanisms (Mohr et al., 2005; Mohr, 2008). The transient type of fatigue that players experience during the most intense game intervals appears to have a relatively short recovery and is suggested to relate physiological mechanisms having a fast recovery rate. Thus a high anaerobic energy turnover with resultant intramuscular accumulation of Hydrogen ions (H+) or inorganic phosphate (Pi) as well as depolarisation of the resting membrane potential induced by disturbances in the muscle Sodium (Na+), Potassium (K+)and Chloride (Cl-) homeostasis have been proposed as main candidates to this type of fatigue (Bangsbo et al., 2006a; Mohr, 2008). In contrast the more permanent type of fatigue mainly accumulating in the second half and especially towards the end of a game has been shown to require longer recovery time. For example has it been shown in several studies that maximal voluntary force capacity is not fully recovered until 72 h after a game in trained football players (Mohr et al., 2005; Mohr 2008; Krustrup et al., 2011). Therefore, it is likely that end-game and post-game fatigue may partly be induced by depleted muscle glycogen stores in individual muscle fibres or in subcellular glycogen compartments with concomitant impairment in muscle calcium (Ca2+ ) handling (Nielsen et al., 2011). In addition, a critical degree of muscle damage potentially negatively affecting numerous neuro-muscular systems may also play a role for long-term fatigue arising in the late stages of a football game (Mohr, 2008; Krustrup et al., 2011).
Thus, the principal aim of fitness training in football is to target the physiological mechanisms causing fatigue and thereby limiting physical football performance. A higher fatigue resistance will allow the players to utilize their technical and tactical capacity throughout a game and especially during the critical game periods. Therefore, the purpose of the present article is to briefly discuss the physiological basis of fatigue resistance training in elite football. Primary focus will be given to speed endurance training and practical examples of individual fitness training in football will be provided.
Training Interventions in Football
Fitness training in any sport has to take account to the demands of the sport, and in team sports such as football the physical demands are multifactorial, which provides an additional challenge to coaches and fitness staff. From a practical point of view it is highly essential to have clear definitions of the fitness training categories. Moreover, these sub-components of a training program have to represent relevant physiological systems of importance for football match performance. Figure 1 shows an overview of the determinants of football performance in relation to different fitness training categories as well as underlying physiological mechanisms being the main targets of these training interventions. The figure is inspired by Bangsbo et al. (2006b).
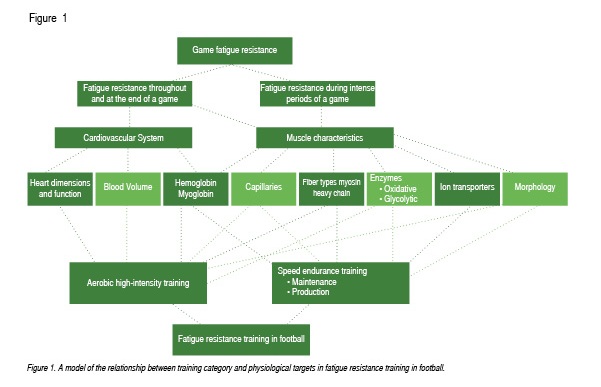
Aerobic high-intensity training
Aerobic training in football is essential for the football endurance capacity and can be divided into aerobic training with low, moderate and high intensity (Figure 1). The two first sub-categories are primarily being utilised as recovery training and maintenance of physical fitness. For example, most technical and tactical sessions will primarily have a physical loading that will classify it as aerobic moderate intensity training. However, aerobic high-intensity training principally aims at improving physiological systems relating to the uptake, transportation and utilization of oxygen; thus, the different components of the cardiovascular system (Bangsbo et al., 2006a; 2006b). Aerobic high intensity training is defined as training at an exercise intensity corresponding to around 90% of the maximal heart rate (Bangsbo et al., 2006b), and in football is usually conducted as specifically designed small-sided games in exercise intervals ranging from 1-4 min separated by 1 min of recovery (Bangsbo et al., 2006b). In one study it was shown that well-trained Scandinavian professional football players increased their VO2Max markedly with concomitant improvements in match performance after a period with additional aerobic high intensity training (Helgerud et al., 2001). The physiological effects from adding aerobic high intensity interval training to already well-trained athletes have in other sports been shown to improve VO2Max, ventilatory and lactate threshold, as well as an increased capacity to engage a higher volume of muscle mass and the ability to oxidize fat relative to carbohydrates (for review see Laursen, 2010). Moreover, football players display improvements in VO2Max (5-11%), running economy (3-7%) and demonstrate lower blood lactate levels during submaximal running after 8-12 wks of aerobic high intensity running (Iaia et al., 2009). Thus, aerobic high intensity training is a highly efficient training protocol for the elite football player. The training conducted in some of the studies mention above was carried out as treadmill running (Helgerud et al., 2001), which normally is not recommended for team sport athletes such as football players due to the lack of specificity of the activity pattern in football and the missing technical and tactical components. Moreover, it has been substantiated that trained football players are able to reach higher heart rates during aerobic high intensity small-sided games compared to generic running intervals, and that similar performance and physiological adaptations can be achieved with small-sided games as treadmill running (Impellizzeri et al., 2006). Thus, specially designed team drills also having a relevant technical and tactical focus are well-suited for as an aerobic high intensity training approach in competitive football (see also Bangsbo et al., 2006b).
Speed endurance training
High intensity training has drawn a lot of attention in during the last decade. In a retrospective study on elite swimmers Mujika et al. (1995) reported that training intensity explained ~45% of the variance in performance improvement over a season with markedly less contribution from training volume and frequency. In a study on Italian football players Ferrari-Bravo et al. (2008) compared traditional aerobic high intensity training (4x4 min at 90-95% of HRMax separated by 3 min recovery) to speed endurance training (3 x 6 maximal shuttle sprints of 40 m). The two different training interventions were added to the normal training twice a week during a period of 7 wks. The study demonstrated that both groups improved their aerobic capacity, but the speed endurance training intervention induced greater improvements in Yo-Yo Intermittent Recovery test, level 1 and repeated sprint ability compared to the aerobic high intensity training protocol. Thus, training intensity appears to play a role for football fitness in trained football players. Anaerobic training is also considered as an essential part of the physical preparation of elite football players in most high level clubs.
Anaerobic training is separated into speed training and speed endurance training with the latter being sub-categorised into speed endurance production and maintenance training (Bangsbo et al., 2006b, see Figure 1). Table 1 shows the training principals in anaerobic training in football.
While speed training is to be performed at maximal exercise intensity speed endurance production and maintenance encompass a wider intensity range, especially speed endurance maintenance training. Beside the differences in range of exercise intensity between speed endurance maintenance and production training there are other important differences. Speed endurance production training has to be performed according to principals relatively similar to speed training only with lower exercise intensity and longer exercise time, but with long recovery periods between the exercise intervals. In contrast speed endurance maintenance training is executed after principals comparable to aerobic high intensity training with relatively short recovery intervals only higher exercise intensity. This means from a practical point of view that speed endurance maintenance training is well-suited as training target in conventional small-sided games (1v1, 2v2 and 3v3). In contrast traditional small-sided games may not be the optimal approach for speed endurance production training where the exercise intensity has to be very high throughout the entire time course of the drill. In a recent study, we compared speed endurance maintenance training conducted as small-sided games (2v2 with goals and goal keepers) to speed endurance production training organised as individual drills (running courses with technical ball challenges, see for example Bangsbo and Mohr, 2014b for drill examples). It was shown the peak and average running speed was ~30 and ~60% higher during the speed endurance production training drills in comparison to the speed endurance maintenance drills. In addition, the players displayed greater improvements in intermittent exercise capacity and repeated sprint ability after 4 wks of the speed endurance production training intervention compared to the speed endurance maintenance training (Mohr and Krustrup, unpublished observations). This is supported by others applying speed endurance production training to youth (Ingebrigtsen et al., 2013) and adults (Thomassen et al., 2010) elite players. IFor example the study by Thomassen et al. (2010) was carried out during a 2 wk period where elite football players lowered their amount of training markedly (total training time was reduced ~30%), but emphasised speed endurance production training combined with aerobic high intensity training. This intervention demonstrated a clear improvement in repeated sprint ability (10 x 20 m sprints), with concomitant muscle molecular adaptations. For example the protein expression of Na+-K+ ATPase α2 subunit and the resting phosphorylation status of the accessory and regulatory FXYD1 protein were significantly increased by intensified training (Thomassen et al., 2010). The Na+-K+ ATPase subunits have been shown to adapt more efficiently to speed endurance production training compared to speed training (Mohr et al., 2007) as well as aerobic training (Iaia et al., 2009) with parallel increases in intense intermittent exercise capacity (for review see Iaia and Bangsbo, 2010) andimproved regulation of interstitial K+ during intense exhaustive exercise (Nielsen et al., 2004). Moreover, the study by Thomassen et al. (2010) indicates that also the resting phosphorylation status of the regulatory FXYD1 subunit in trained football players can be changed with short-term prioritisation of speed endurance training(Thomassen et al., 2010). This is suggested to elevate the muscle Na+-K+ ATPase activity at the onset of exercise, which may contribute to improved fatigue resistance during repeated intense exercise such as the aforementioned peak intensity periods of a game where players experience temporary fatigue. In addition to the clear scientific evidence regarding up-regulation of the Na+-K+ ATPase potential after speed endurance production training, other sarcolemmal transport proteins have also been shown to respond the this type of training. Indeed the Na+/H+ exchanger isoform 1 (NHE1) and to a lesser degree the mono-carboxylate transporter (MCT) have been shown to increase after speed endurance training in already trained athletes (Iaia and Bangsbo, 2010; see Table 2). Thus, speed endurance training improves the capacity to regulate intramuscular pH, which may directly improve fatigue resistance during the high intensity periods of a game. An increased Na+/H+ exchange protein may also elevate the Na+ uptake inside the muscle cell and thereby potentially further stimulate the Na+-K+ ATPase activity and cause a hyperpolarization of the resting membrane potential (Iaia and Bangsbo, 2010).
The physiological response to a standardised speed endurance training session was investigated in a study by Mohr et al. (2007) where participants completed eight 30 s runs at an exercise intensity corresponding to ~130% VO2Max with 150 s recovery. It was shown that blood lactate and plasma K+ reached peak levels >16 and >6 mmol·L-1, respectively, while muscle lactate rose and muscle pH declined markedly (Mohr et al., 2007). Thus, the muscle ion transport systems (for example Na+-K+ ATPase, NHE1 and MCT) are highly stimulated during the speed endurance and high intensity training regimes, which is supported by clear improvements in fatigue resistance during repeated high intensity exercise and concomitant increases in the protein expression of the respective ion transporters mention above as well as improved muscle ion regulation and buffer capacity. Table 2 provides a comprehensive summary of the change in high intensity exercise performance (Table 2A) and muscle ion transport proteins playing key roles in regulating intramuscular pH and ion homeostasis (Table 2B) after periods of different high intensity training interventions having speed endurance training elements.
Intensified training and endurance performance
Several studies applying speed endurance based training regimes have studied the effects on physiological variables associated with performance in endurance based sports events. Studies using moderately trained subjects have demonstrated improvements ranging between 3-7% in VO2Max (Iaia and Bangsbo, 2010), as well as increased VO2Max speed (Esfarjani and Laursen, 2007). In a study by Bangsbo et al. (2009) well-trained distance runners demonstrated a ~3% increase in 3 and 10 km running performance as well as maintenance of VO2Max and both glycolytic and oxidative enzyme activity. However, running economy at continuous submaximal speeds improved by 3% and RER during fast speed running was significantly lowered indicated a higher fat oxidation capacity. This may exert a muscle glycogen sparing effect during prolonged exercise, which is supported by others showing a decrease in muscle glycogen degradation after a period with speed endurance training (Iaia et al., 2009). Moreover, it has been shown in elite football players that a short period with lowered training volume, but increased focus on speed endurance training, caused improvements in running economy (Christensen et al., 2011). Thus, speed endurance training seems also to improve mechanical efficiency during exercise in trained footballers, while the aerobic power and muscle oxidative capacity can be maintained. These adaptations may contribute to elevated fatigue resistance throughout and especially at in the final stage of a football game.
Yet another physiological advantage of intensified training in football is to ensure that a greater portion of the muscle is being trained. For example, has it been shown in runners after reducing their training volume and raising the training intensity that the area of the type IIx fibres was higher (Iaia et al., 2009). The muscle fibre type recruitment is highly dependent on exercise intensity and mode. Based on studies which have observed decrements in muscle glycogen in both type I and II fibres, we can assume that all fibre types are being used during a football game (Krustrup et al., 2006). Thus, by including aerobic high intensity and speed endurance training in your training strategy and more homogenous training response in all muscle fibre types may be expected.
Individual training in football
It is necessary in team sports such as football to plan and organise the fitness training in relation to the individual demands of all the players in the team. Match analysis studies demonstrate large individual differences between the players in total distance covered, high intensity running (Mohr, 2008; Mohr et al., 2003), as well as accelerations and sprint characteristics (Mohr, 2008; DiSalvo et al., 2009; Bangsbo and Mohr, 2014). Figure 2 shows the peak speed, length and duration of all sprints performed in a game by two different players (A and B). It is clear that player A performs markedly more sprinting during the game and several sprints are longer than 30 m and a peak speed higher than 30 km·h-1 is reached. In contrast player B performed only few sprints whereas most are short accelerations.
To a certain degree these differences are related to the playing position of the player, but also within the same playing position large differences are present (see for example Bangsbo and Mohr, 2014). In addition, similar variations are present in small-sided games (Dellal et al., 2012), and with some player types there is not consensus between the work profile in games and the training response during small-sided games (Mohr, unpublished observations). Thus, these players may not be sufficiently physically loaded during small-sided games to cope with their individual game demands. Therefore, fitness training in high level football is proposed to include an individual player-specific approach. Some top class teams have to our knowledge and experience player-specific fitness training as a regular part of their training model. Figure 3 shows an example of a speed endurance production drill used by a top-class team. The drill was constructed for a top-class attacker after detailed analysis of the game activity profile of the player including important technical challenges that were deemed as key performance indicators for the specific player. In the drill the players performs brief accelerations (~5 m), explosive directional changes and longer sprints (~30 m), which are similar to runs the players performs in important game situations (Figure 3). The player has a number of technical challenges including three shots at the goal (Figure 3). These are all similar to game situations for the respective player and are all performed at high speed, while the player is under physical stress.
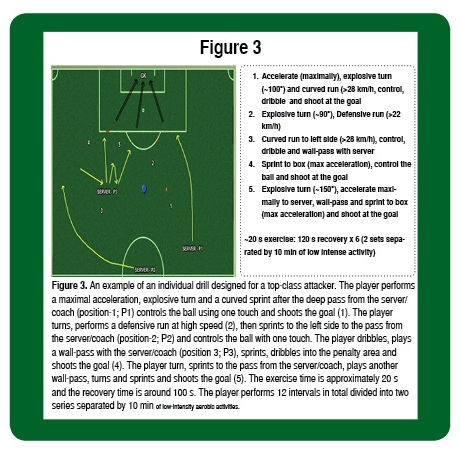
The drill shown above is constructed to full-fill the criteria of speed endurance production training with a very high intensity throughout the drill. The running speed is adjusted to peak speeds reached in a game based on match analysis data and can be monitored during the drill with for example real time GPS technology. Finally, the technical football challenges are part of the key performance indicators of the specific player which partly are determined by the coaches and partly by detailed video analysis. From a physiological perspective, the player is training systems associated with fatigue resistance during the demanding peak periods of a game and from a football perspective the player is being challenged within individual technical key components of performance in physically demanding conditions. From a motivational and educational point of view this type of training also has advantages in order to make the player aware of his/hers own game demands and physical development.
Summary
In summary, football match-play is highly demanding and exerts a considerable taxation of aerobic and anaerobic energy systems, which has been demonstrated to provoke fatigue during and at the end of a game. Therefore, a principal aim of physical training in football is to improve physiological systems associated with game-specific fatigue resistance. Aerobic high intensity and speed endurance training are suggested as main components of fatigue-resistance training in football, and have been demonstrated to be targeting a wide range of physiological systems linked to improved football fatigue resistance. Finally, large individual variations are present between the players in a team in physical and technical demands. Therefore, it is proposed that individual player-specific fitness training is incorporated into training regimes in elite football.
Acknowledgement
The present paper is dedicated in memory of our late friend and colleague, Nick Broad.
References
Bangsbo, J., Mohr, M., and Krustrup, P. (2006a). Physical and metabolic demands of training and match-play in the elite football player. J. Sports Sci. 24:665-674.
Bangsbo, J., Mohr, M., Poulsen, A., Perez-Gomoz, J., and Krustrup, P. (2006b). Training and testing the elite athlete. J. Exerc. Sci. Fit. 1:1-14.
Bangsbo, J., Gunnarsson, T.P., Wendell, J., Nybo, L., Thomassen, M. (2009). Reduced volume and increased training intensity elevates Na+-K+ pump α2-subunit expression as well as short- and long-term work capacity in humans. J. Appl. Physiol. 107:1771-1780.
Bangsbo. J. Physiological Demands of Football. (2014a) Sports Science Exchange. Vol. 27, No. 125, 1-6.
Bangsbo, J., and Mohr, M. (2014b). Individual Training in Football. Bangsbosport, Denmark ISBN 9788799488025.
Christensen, P.M., Krustrup, P., Gunnarsson, T.P., Kiilerich, K., Nybo, L., and Bangsbo, J. (2011). VO2 kinetics and performance in soccer players after intense training and inactivity. Med. Sci. Sports Exerc. 43:1716-1724.
Dellal, A., Drust, B., and Lago-Penas, C. (2012). Variation of activity demands in small-sided soccer games. Int. J. Sports Med. 33:370-375.
Di Salvo, V., Gregson, W., Atkinson, G., Tordoff, P., and Drust, B. (2009). Analysis of high intensity activity in Premier League soccer. Int. J. Sports Med. 30:205-212.
Esfarjani, F. and Laursen, P.B. (2007). Manipulating high-intensity interval training: effects on VO2max, the lactate threshold and 3000 m running performance in moderately training males. J. Sci. Med. Sport. 10:27-35.
Ferrari-Bravo, D., Impellizzeri, F.M., Rampinini, E., Castagna, C., Bishop, D., and Wisloff, U. (2008). Sprint vs. interval training in football. Int. J. Sports Med. 29:668-674.
Helgerud, J., Engen, L.C., Wisloff, U., and Hoff, J. (2001). Aerobic endurance training improves soccer performance. Med. Sci. Sport Exerc. 33:1925-1931.
Iaia, F.M., Hellsten, Y., Nielsen, J.J., Fernström, M., Sahlin, K., and Bangsbo, J. (2009). Four weeks of speed endurance training reduces energy expenditure during exercise and maintains muscle oxidative capacity despite a reduction in training volume. J. Appl. Physiol. 106:73-80.
Iaia, F.M., and Bangsbo, J. (2010). Speed endurance training is a powerful stimulus for physiological adaptations and performance improvements of athletes. Scand. J. Med. Sci. Sports. 20:11-23.
Impellizzeri, F.M., Marcora, S.M., Castagna, C., Reilly, T.P., Sassi, A., Iaia, F.M., and Rampinini, E. (2006). Physiological and performance effects of generic versus specific aerobic training in soccer players. Int. J. Sport Med. 27:483-492.
Ingebrigtsen, J., Shalfawi, S.A., Tønnessen, E., Krustrup, P., and Holtermann, A. (2013). Performance effects of 6 weeks of anaerobic production training in junior elite soccer players. J. Strength Cond. Res. 27:1861-1867.
Krustrup, P., Mohr, M., Steensberg, A., Bencke, J., Kjaer, M., and Bangsbo, J. (2006). Muscle and blood metabolites during a soccer game: implications for sprint performance. Med. Sci. Sports Exerc. 38:1165-1174.
Krustrup, P., Ortenblad, N., Nielsen, J., Nybo, L., Gunnarsson, T.P., Iaia, F.M., Madsen, K., Stephens, F., Greenhaff, P., and Bangsbo. J. (2011). Maximal voluntary contraction force, SR function and glycogen resynthesis during the first 72 h after a high-level competitive soccer game. Eur. J. Appl. Physiol. 111:2987-2995.
Laursen, P.B. (2010). Training for intense exercise performance: high-intensity or high-volume training? Scand. J. Med. Sci. Sports. Suppl. 2:1-10.
Mohr, M., Krustrup, P., and Bangsbo, J. (2003). Match performance of high-standard soccer players with special reference to development of fatigue. J. Sports Sci. 21:519-528.
Mohr, M., Krustrup, P., and Bangsbo, J. (2005). Fatigue in soccer: A brief review. J. Sport Sci. 23:593-99.
Mohr, M., Krustrup P., Nielsen J.J, Nybo L., Rasmussen M.K., Juel C., and Bangsbo J. (2007). Effect of two different intense training regimes on skeletal muscle ion transport systems and fatigue development. Am. J. Physiol. Reg. Intergr. Comp. Physiol. 292:R1594-R1602.
Mohr. M. (2008). Fatigue development in soccer with reference to intense intermittent exercise. Copenhagen, Denmark, ISBN 9878791771163.
Mujika, I., Chatard, J.C., Busso, T., Geyssant, A., Barala, F., and Lacoste, L. (1995). Effects of training on performance in competitive swimming. Can. J. Appl. Physiol. 20:395-406.
Nielsen, J.J., Mohr, M., Klarskov, C., Juel, C., and Bangsbo, J. (2004). Effect of high-intensity intermittent training on potassium kinetics and performance in humans. J. Physiol. 554:857-870.
Nielsen, J., Krustrup, P., Nybo, L., Gunnarsson, T.P., Madsen, K., Schrøder, H.D., Bangsbo, J., and Ortenblad, N. (2011). Skeletal muscle glycogen content and particle size of distinct subcellular localizations in the recovery period after a high-level soccer match. Eur. J. Appl. Physiol. 112:3559-3567.
Rampinini, E., Impellizzeri, F. M., Castagna, C., Coutts, A. J., and Wisloff, U. (2009). Technical performance during soccer matches of the Italian Serie A league: effect of fatigue and competitive level. J. Sci. Med. Sport. 12:227-33.
Thomassen, M., Christensen, P.M., Gunnarsson, T.P., Nybo, L., & Bangsbo, J. (2010). Effect of 2-wk intensified training and inactivity on muscle Na+-K+ pump expression, phospholemman (FXYD1) phosphorylation, and performance in soccer players. J. Appl. Physiol. 108:898-905.