KEY POINTS
- The gastrointestinal (GI) tract plays a critical role in delivering carbohydrate and fluid to the blood during prolonged exercise, and can therefore be a major determinant of performance.
- GI problems are common in endurance athletes and might be prevented by adapting the gut during training to the specific conditions it will be exposed to in competitions.
- “Training the gut” can improve stomach comfort and reduce symptoms of GI distress.
- There is a substantial body of evidence to support the fact that the GI tract is highly adaptable.
- The GI tract, and the transport proteins within it, adapt with changes in diet in a nutrient-specific way.
- So-called “nutritional training” can improve gastric emptying and absorption, and likely reduce the chances and/or severity of GI problems, thereby improving endurance performance and providing a better experience for the athlete.
INTRODUCTION
The functioning of the gastrointestinal (GI) tract can have a major effect on endurance performance and there are two main reasons for this, 1) the GI tract is responsible for delivering carbohydrate and fluid to the blood during prolonged exercise and it has been demonstrated that the delivery of fluids and carbohydrate can delay fatigue and enhance endurance performance, and 2) there is a high incidence of Gl problems in athletes participating in endurance events, suggesting that GI function may be compromised in some athletes in those conditions.
The importance of the GI tract is often underestimated by athletes. A lot of time is spent on thinking about how to prepare skeletal muscles for competition, whereas little or no time is spent thinking about conditioning the GI tract. Especially during prolonged exercise, the supply of exogenous fluid and carbohydrates sources can be critical to performance (Jeukendrup, 2011). In addition, GI symptoms such as bloating, cramping, diarrhea and vomiting are common in many sports, especially in endurance sports (de Oliveira et al., 2014). Without a well-functioning GI system, delivery of nutrients will be impaired and a range of GI symptoms may develop.
It is clear that the intestinal tract is a highly adaptable organ and it has been suggested that targeted training of the intestinal tract may improve the delivery of nutrients during exercise, while at the same time alleviating some (or all) of the symptoms of GI distress (Jeukendrup & McLaughlin, 2011). This training is sometimes referred to as “training the gut” and has received relatively little attention in the literature. This Sports Science Exchange article will provide a brief overview of the evidence suggesting that the GI system can adapt to nutritional training and discuss how this knowledge might be used in practice.
GASTRIC EMPTYING
The first barrier for delivering exogenous carbohydrate and fluids to the blood and ultimately the contracting muscles is the stomach. The stomach is the gatekeeper to the intestine, and gastric emptying will determine fluid and nutrient delivery to the intestine. Anecdotally, athletes complain about drinks accumulating in the stomach and feeling bloated, especially during high intensity or very prolonged exercise in hot conditions (Neufer et al., 1989). Dehydration can contribute to this phenomenon and make symptoms worse (Neufer et al., 1989; Rehrer et al., 1990). There is also anecdotal evidence that the stomach can adapt to ingesting large volumes of fluid, solids or combinations. For example, serious contestants in eating competitions are known to “train” their stomach to hold larger volumes of food with less discomfort and through regular training are able to eat volumes of food within a small time window that are unthinkable for the average and untrained person. The current all-time record is 69 hot dogs (with bun) in 10 min. In order to achieve this, competitive eaters train using a variety of methods: chewing large pieces of chewing gum for longer periods of time, stomach extension by drinking fluids or by eating the competition foods. Volumes are progressively increased and it takes many weeks to reach a level where these eaters can be competitive. This demonstrates the adaptability of the stomach. Conducting this “stomach training” has two main effects, 1) the stomach can extend and contain more food, and 2) a full stomach can be tolerated better and is not perceived as full. Both aspects could be relevant to an exercise situation.
Current guidelines recommend fluid intakes during exercise that prevent 2% of body mass loss (mild dehydration). Especially in trained athletes and hot conditions, when sweat rates are high, recommended fluid intake can be high. Such high intakes can cause stomach discomfort and in some cases other GI problems. Therefore, athletes are generally managing GI comfort on the one hand and fluid plus carbohydrate delivery on the other hand. We have recommended to train with these higher intakes so there is less discomfort and a reduced chance of GI distress (Jeukendrup, 2013; 2014; Jeukendrup & McLaughlin, 2011). However, there are very few studies that have directly investigated the effects of “nutritional training of the stomach.”
Lambert et al. (2008) asked a group of runners to run five times while ingesting a carbohydrate-electrolyte solution at a rate approximately equal to their sweat rates during 90 min at 65% VO2max in a ~ 25 oC, 30% relative humidity (RH) environment. This was a large volume of fluid that caused a lot of discomfort during their first run. Interestingly, they observed that stomach comfort significantly improved over time by practicing these high intakes and by the fourth and fifth runs, the runners were able to comfortably tolerate the large volumes.
It must be noted that this improved comfort occurred without measurable changes in the rate of gastric emptying (Lambert et al., 2008). Perhaps the stomach adapted by extending the stomach walls, allowing greater space for fluid. This would likely reduce feelings of stomach discomfort and reduce the stimulus for faster gastric emptying. Especially for those athletes who experience GI discomfort even when ingesting relatively small volumes, training the intake of larger volumes could be an effective strategy to avoid these problems in races.
Studies have also demonstrated that gastric emptying of carbohydrate can be accelerated by increasing the dietary intake of that carbohydrate. Cunningham et al. (1991) supplemented the diet of two groups of volunteers with 400 g of glucose/day for three days. The time to empty half of the glucose in the glucose test meal was significantly faster after the standard diet had been supplemented with glucose compared with the standard diet alone (median (range) 20.7 (4.6-36.8) vs. 29.1 (19.8-38.4) min). Interestingly the gastric emptying of a protein drink was unchanged (median (range) 18.0 (12.5-23.6) vs. 16.1 (9.6-22.7) min). The authors concluded that rapid and specific adaptation of the small intestinal regulatory mechanisms for gastric emptying of nutrient solutions can occur in response to increases in dietary load. Another study demonstrated that supplementing a standard diet with 440 g glucose/day for four to seven days accelerated gastric emptying of both glucose and fructose (half-time of 82 ± 8 vs. 10 6 ±10 min for glucose and 73 ± 9 vs. 106 ± 9 min for fructose) (Horowitz et al., 1996). A third, more recent study showed that daily ingestion of 120 g of fructose for three days accelerated gastric emptying of fructose but not of glucose (Yau et al., 2014). It appears that the relatively short duration of the dietary manipulation (three days) in these studies was sufficient to cause adaptations in gastric emptying.
In summary, specific nutritional challenges result in specific adaptations of gastric emptying to that challenge and effects have been observed after only three days of dietary manipulations. While very few studies have specifically trained the gut to improve tolerance and gastric emptying during exercise, in those that have the results generally look promising.
INTESTINAL SUGAR TRANSPORT
Once emptied from the stomach, most fluid and sugar absorption will take place in the duodenum and jejunum. Fluids will follow an osmotic gradient, but sugars need to be transported across the epithelial membrane of the intestine. The monosaccharides glucose and galactose are transported across the luminal membrane of enterocytes by the sodium dependent glucose transporter SGLT1 (Figure 1). Absorption of glucose (and galactose) is coupled to sodium transport and the associated electrochemical gradient. A Na/K+ ATP-ase, located at the basolateral membrane is responsible for maintaining the electrochemical gradient.
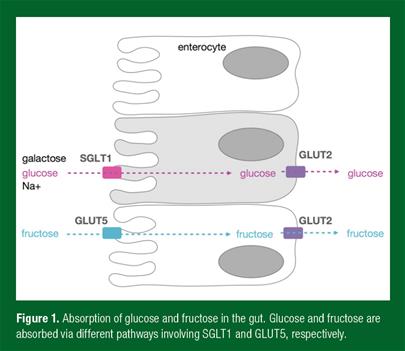
The absorption of the monosaccharide fructose takes place through a different transporter (GLUT5) that is not sodium dependent and is highly specific to fructose. From the enterocyte to the systemic circulation, the sugars need to pass through the basolateral membrane. To achieve this, all three monosaccharides use the bidirectional transporter GLUT2 which is also sodium independent. The capacity of GLUT2 to transport glucose across a concentration gradient is believed to be very large (Kellett et al., 2008). Therefore, SGLT1 and GLUT5 are the most important transporters with respect to carbohydrate absorption, and their abundance and activity will determine the absorption rates of monosaccharides.
CARBOHYDRATE TRANSPORTERS AND GLUCOSE TRANSPORT DURING EXERCISE
Regulation of these transport proteins is essential for the provision of glucose to the body in resting conditions. Also during exercise, when exogenous delivery of carbohydrate may be important for performance, the transporters will be responsible for glucose delivery to the working muscle. Exercise studies have provided indirect, but strong evidence that the delivery of carbohydrate is limited by the transport capacity of SGLT1 (for reviews see Jeukendrup, 2011; 2013; 2014; Jeukendrup & McLaughlin, 2011).
At ingestion rates over 60-70 g of carbohydrate/hour (glucose, sucrose, maltose, maltodextrin, starch), exogenous carbohydrate oxidation peaks at ~60 g/hour (Jeukendrup, 2011; 2013; 2014; Jeukendrup & McLaughlin, 2011) and this is thought to be because the SGLT1 transporters become saturated. Because fructose uses a different transport mechanism, the combined intake of glucose and fructose can result in much higher exogenous oxidation rates (Jentjens et al., 2004). There also appears to be a dose response relationship between carbohydrate intake and performance (Smith et al., 2010; 2013; Vandenbogaerde & Hopkins, 2011). This coupled with the likelihood that a relatively low capacity of the intestine to absorb carbohydrate, in combination with a high carbohydrate intake, may result in GI distress (de Oliveira et al., 2014). The search for ways to increase the capacity to absorb carbohydrate has continued. Training the gut has been proposed as a method to increase SGLT1 transporter number and/or activity, but evidence in humans, thus far, is limited (Jeukendrup, 2013).
REGULATION OF INTESTINAL GLUCOSE TRANSPORT
In order to develop practical recommendations, it is important to understand the regulation of intestinal glucose transport. The regulation of glucose absorption has been shown to be directly linked to the expression of SGLT1 protein. Dr. Bob Crane proposed the existence of a Na+/glucose co-transporter in 1960 at the Symposium on “Membrane Transport and Metabolism” in Prague (Kleinzeller, 1961) but the actual transporter was not identified until the early 1980s (Hosang et al., 1981). Studies in the 1960s also observed that dietary carbohydrate intake can influence the capacity to absorb glucose (Ginsburg & Heggeness, 1968). In 1983, it was demonstrated that intestinal transporters were upregulated and downregulated depending on dietary composition (Karasov et al., 1983). Work with rodents suggested that dietary changes did not have to be extreme in order to observe effects on absorption, that the effects were seen for sugars and also amino acids, and that increases in absorption were observed in as little as 0.5 days (Karasov et al., 1983).
When sugar transporters were identified in the gut in the 1980s, studies started to measure changes in SGLT1 content and activity in response to diet. Both the activity and abundance of SGLT1 have been shown to be regulated by dietary carbohydrate intake in a number of rodent models (Dyer et al., 2009). It is clear that SGLT1 protein responds to glucose concentrations in the lumen. However, when membrane-impermeable glucose analogues were used, SGLT1 was stimulated to the same degree (Dyer et al., 2009). This suggested that a glucose sensor detects glucose or its analogues, initiating the upregulation of the SGLT1 transporters.
TIME COURSE OF TRANSPORTER ADAPTATION
In mice, intestinal SGLT1 protein in brush-border membrane vesicles in the mid small intestine increased 1.9-fold after two weeks of a high carbohydrate diet (Margolskee et al., 2007). A study in horses, which are believed to be slow adapters to increases in carbohydrate intake, reported that SGLT1 protein expression from intestinal biopsies was increased after just one week of high carbohydrate feeding and the abundance increased further after one and two months on the diet (Dyer et al., 2009). In piglets who received a high carbohydrate diet for three days, increases of SGLT1 protein as well as glucose absorption were demonstrated (Moran et al., 2010).
Although no direct human studies exist, a large number of animal studies suggest that the time course of changes in SGLT1 expression is relatively rapid. Several studies have observed significant changes after only a few days of dietary change. It seems therefore reasonable to suggest that several days of a high carbohydrate intake can increase SGLT1 content and the capacity to absorb glucose, but more prolonged exposure to the diet could result in greater adaptations.
REGULATION OF CARBOHYDRATE ABSORPTION IN ATHLETES
The vast majority of evidence presented so far has come from animal studies. However, there is also some compelling evidence in humans. An elegant study by Cox et al. (2010) gave us the most important clues that diet manipulation can result in improved delivery of carbohydrate during exercise. Sixteen endurance-trained cyclists were divided into a high carbohydrate group and a control group. For 28 days both groups trained (16 h/week) and their performance improved as a result of this training. During this time, both groups received a diet with a moderate carbohydrate content (5 g/kg/day). The high carbohydrate group was supplemented with an additional 1.5 g/kg per hour of exercise performed daily and this carbohydrate supplement was provided mainly in the form of a glucose drink. In addition, they received carbohydrate-rich foods to meet the hourly demands of exercise. The control group also received a nutritional supplement but this was composed of fat and protein-rich foods with limited carbohydrate content. Subjects in the high carbohydrate group consumed the supplements before and during exercise as well as immediately after exercise. The cyclists in the control group consumed their supplement after exercise. On average the carbohydrate-supplemented group had a high daily carbohydrate intake of 8.5 g/kg whereas the control group consumed 5.3 g/kg/day.
Before and after the 28 day training period all subjects performed an exercise trial at the same intensity in which they received a 10% carbohydrate solution (Cox et al., 2010). Isotopic tracers were used to measure the oxidation of the exogenous carbohydrate. It was observed that exogenous carbohydrate oxidation was improved after the carbohydrate-supplemented diet. The most likely explanation is an increase in the ability to absorb carbohydrate as a result of an upregulation of SGLT1 transporters. It was concluded that, for athletes who compete in endurance events in which exogenous carbohydrate is an important energy source and there is ample opportunity to ingest carbohydrate, this higher carbohydrate intake approach may be beneficial (Cox et al., 2010; Jeukendrup, 2013; 2014).
It has become clear that an increase in dietary carbohydrate intake can increase the abundance and activity of intestinal SGLT1 transporters and that this results in an improved capacity to absorb carbohydrate. The reverse may be true as well. With carbohydrate restriction through reducing carbohydrate intake, high fat or even ketogenic diets, or by reducing total energy intake, the daily carbohydrate intake can become very low which may have attenuating effects on gastric emptying and intestinal absorption.
GASTROINTESTINAL PROBLEMS
Gastrointestinal problems are very common among athletes, and 30-50% of all athletes experience such problems regularly (de Oliveira et al., 2014). The causes are still largely unknown but appear to be partly genetically determined and highly individual. The mechanisms are likely to be different for upper and lower GI problems. Symptoms are also more likely to occur and are exacerbated by hot weather conditions and dehydration (de Oliveira et al., 2014). Although a link is not always apparent with nutritional intake, certain practices have been found to correlate with the incidence of GI problems, with fiber intake, fat intake and highly concentrated carbohydrate solutions appearing to increase the prevalence of GI problems.
Different GI problems may have different causes but it is generally thought that, 1) a bloated feeling is related to reduced gastric emptying during prolonged exercise, 2) diarrhea is a result of large osmotic shifts, and 3) many GI problems may be related to a redistribution of blood flow, where blood is diverted away from the gut to muscle and skin. It is thought that “training the gut” may alleviate or alter this physiology and reduce some of the associated symptoms, perhaps by improving gastric emptying, the perception of fullness (reduced bloating), improved tolerance of larger volumes and more rapid absorption causing less residual volume and smaller osmotic shifts.
PRACTICAL RECOMMENDATIONS AND CONCLUSIONS
A summary of practical implications is depicted in Figure 2. Some extrapolations from animal studies need to be made, but it is likely that adaptations in the human intestine are as rapid as those seen in other mammals. This means that several days and certainly two weeks of a high carbohydrate diet would result in significant increases in the SGLT1 content of the intestinal lumen. Based on animal data, an increase in dietary carbohydrate from 40% to 70% could result in a doubling of SGLT1 transporters over a period of two weeks. We would not necessarily recommend to have a high carbohydrate intake every day and thus adaptation periods may need to be longer. Until we have more firm data, it is suggested that training with high carbohydrate intake once a week will improve tolerance within 6-10 weeks.
In addition to an increased absorptive capacity it is essential to make sure that higher carbohydrate intakes can be tolerated and are also emptied from the stomach. Although it is generally believed that gastric emptying is not a limiting factor, it is likely that when a combination of factors (for example heat, high carbohydrate intake and high intensity exercise) all act together, gastric emptying may be compromised. Therefore, it is important to practice a race nutritional strategy in training and get used to higher volumes of intake or higher carbohydrate intakes.
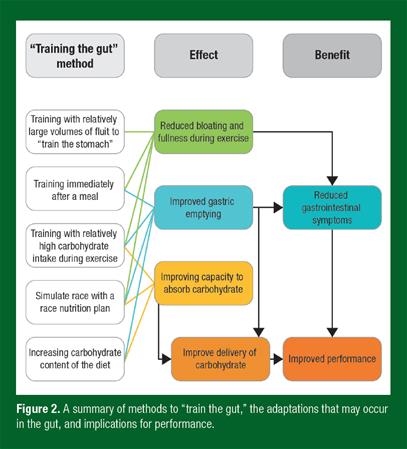
When athletes are carbohydrate restricting, following a high fat or ketogenic diet or are reducing energy intake, in order to lose weight, the reduced daily carbohydrate load may reduce the capacity to absorb carbohydrates during competition. This could be a reason why these athletes anecdotally seem to report more GI problems. These athletes would be advised to include some high carbohydrate days in their training as well.
Current guidelines suggest that for exercise lasting for up to two hours, a carbohydrate intake up to about 60 g/h is recommended. When the exercise lasts two hours or more, slightly greater amounts of carbohydrate (90 g/h) would be recommended and these carbohydrates should consist of a mix of multiple transportable carbohydrates (e.g., glucose:fructose or maltodextrin:fructose). In order to obtain a carbohydrate intake of 90 g/h athletes could “mix and match” to fulfill their personal preferences and take into account their tolerance (Jeukendrup, 2013; 2014).
Since the gut is highly adaptable, it seems wise to regularly (at least once a week) ingest carbohydrate during exercise and mimic a feeding strategy that would be used in competition. The gut will be “trained” to absorb more carbohydrate, resulting in higher rates of exogenous carbohydrate oxidation, less GI distress and most likely better performance.
References
Cox, G.R., S.A. Clark, A.J.Cox, S.L. Halson, M. Hargreaves, J.A. Hawley, N. Jeacocke, R,J, Snow, W.K. Yeo, and L.M. Burke (2010). Daily training with high carbohydrate availability increases exogenous carbohydrate oxidation during endurance cycling. J. Appl. Physiol. 109: 126-134.
Cunningham, K.M., M. Horowitz, and N.W. Read (1991). The effect of short-term dietary supplementation with glucose on gastric emptying in humans. Br. J. Nutr. 65: 15-19.
de Oliveira, E.P., R.C. Burini, and A.E. Jeukendrup (2014) Gastrointestinal complaints during exercise: prevalence, etiology, and nutritional recommendations. Sports Med. 44: S79-S85.
Dyer, J., Al-Rammahi M, Waterfall L, Salmon KS, Geor RJ, Bouré L, Edwards GB, Proudman CJ, Shirazi-Beechey SP (2009). Adaptive response of equine intestinal Na+/glucose co-transporter (SGLT1) to an increase in dietary soluble carbohydrate. Pflugers Arch 458, 419-430.
Ginsburg, J.M., and F.W. Heggeness (1968). Adaptation in monosaccharide absorption in infant and adult rats. J. Nutr. 96: 494-498.
Horowitz, M., K.M. Cunningham, J.M. Wishart, K.L. Jones, and N.W. Read (1996). The effect of short-term dietary supplementation with glucose on gastric emptying of glucose and fructose and oral glucose tolerance in normal subjects. Diabetologia 39: 481-486.
Hosang, M., E.M. Gibbs, D.F. Diedrich, and G. Semenza (1981). Photoaffinity labeling and identification of (a component of) the small-intestinal Na+,D-glucose transporter using 4-azidophlorizin. FEBS Lett. 130: 244-248.
Jentjens, R.L., J. Achten, and A.E. Jeukendrup (2004). High oxidation rates from combined carbohydrates ingested during exercise. Med. Sci. Sports Exerc. 36: 1551-1558.
Jeukendrup, A.E. (2011). Nutrition and endurance sports: running, cycling, triathlon. J. Sports Sci. 29: S91-S99.
Jeukendrup, A.E. (2013). The new carbohydrate intake recommendations. Nestle Nutr. Inst. Workshop 75: 63-71.
Jeukendrup, A.E. (2014). A step towards personalized sports nutrition: carbohydrate intake during exercise. Sports Med. 44: S25-S33.
Jeukendrup, A.E., and J. McLaughlin (2011). Carbohydrate ingestion during exercise: effects on performance, training adaptations and trainability of the gut. Nestle Nutr. Inst. Workshop 69: 1-12. (discussion 13-17).
Karasov, W.H., R.S. Pond 3rd, D.H. Solberg, and J.M. Diamond (1983). Regulation of proline and glucose transport in mouse intestine by dietary substrate levels. Proc. Natl. Acad. Sci. 80: 7674-7677.
Kellett, G.L., E. Brot-Laroche, O.J. Mace, and A. Leturque (2008). Sugar absorption in the intestine: the role of GLUT2. Annu. Rev. Nutr. 28: 35-54.
Kleinzeller A, and A. Kotyk (eds.) (1961). Membrane transport and metabolism. Prague: Academy of Sciences.
Lambert, G.P., J. Lang, A. Bull, J. Eckerson, S. Lanspa, and J. O'Brien (2008). Fluid tolerance while running: effect of repeated trials. Int. J. Sports Med. 29: 878-882.
Margolskee, R.F., J. Dyer, Z. Kokrashvili, K.S. Salmon, E.Ilegems, K. Daly, E.L. Maillet, Y. Ninomiya, B. Mosinger, and S.P. Shirazi-Beechey (2007). T1R3 and gustducin in gut sense sugars to regulate expression of Na+-glucose cotransporter Proc. Natl. Acad. Sci..104: 15075-15080.
Moran, A.W., M.A. Al-Rammahi, D.K. Arora, D.J. Batchelor, E.A. Coulter, C. Ionescu, D. Bravo, and S.P. Shirazi-Beechey (2010). Expression of Na+/glucose co-transporter 1 (SGLT1) in the intestine of piglets weaned to different concentrations of dietary carbohydrate. Br. J. Nutr. 104: 647-655.
Neufer, P.D., A.J. Young, A.J. and M.N. Sawka (1989). Gastric emptying during exercise: effects of heat stress and hypohydration. Eur. J. Appl. Physiol. 58: 433-439.
Rehrer, N.J., E.J. Beckers, F. Brouns, F. ten Hoor, and W.H.M. Saris (1990). Effects of dehydration on gastric emptying and gastrointestinal distress while running. Med. Sci. Sports Exerc. 22: 790-795.
Smith, J.W., J.J. Zachwieja, F. Péronnet, D.H. Passe D, Massicotte, C. Lavoie, and D.D. Pascoe (2010). Fuel selection and cycling endurance performance with ingestion of [13C]glucose: evidence for a carbohydrate dose response. J. Appl. Physiol. 108: 1520-1529.
Smith, J.W., D.D. Pascoe, D.H. Passe, B.C. Ruby, L.K. Stewart, L.B. Baker, and J.J. Zachwieja (2013). Curvilinear dose-response relationship of carbohydrate (0-120 g.h-1) and performance. Med. Sci. Sports Exerc. 45: 336-341.
Vandenbogaerde, T.J., and W.G. Hopkins (2011). Effects of acute carbohydrate supplementation on endurance performance: a meta-analysis. Sports Med. 41: 773-792.
Yau, A.M., J. McLaughlin, R.J. Maughan, W. Gilmore, and G.H. Evans (2014). Short-term dietary supplementation with fructose accelerates gastric emptying of a fructose but not a glucose solution. Nutrition 30: 1344-1348.