KEY POINTS
-
Heat acclimatization (or acclimation) confers biological adaptations that reduce physiological strain (e.g., heart rate and body temperature), improve comfort, improve exercise capacity and reduce the risks of serious heat illness during exposure to heat stress.
-
The biological adaptations include integrated thermoregulatory, cardiovascular, fluid-electrolyte, metabolic and molecular responses.
-
Heat acclimatization occurs when repeated exercise-heat exposures are sufficiently stressful to invoke profuse sweating and elevate whole-body temperatures.
-
Generally, about 1-2 wk of ~90 min daily heat exposures are required; but highly aerobic fit athletes can heat acclimatize.
-
Heat acclimatization is specific to the climatic heat stress (desert or tropic) and physical exercise intensities the athletes are exposed to, which should simulate the expected competitive environment.
INTRODUCTION
If given sufficient time to adapt, and access to shade and adequate water, healthy persons can tolerate extended exposure to virtually any naturally occurring environmental heat stress (Sawka et al., 1996). Heat stress results from the interaction of environmental conditions (temperature, humidity, solar radiation), physical work rate (body heat production) and wearing of heavy clothing/equipment that impedes heat loss (Gagge & Gonzalez, 1996; McLellan et al., 2013; Sawka et al., 1996). Environmental heat stress and physical exercise interact synergistically to increase strain on physiological systems (Sawka et al., 2011). Thus, conducting physical exercise in warm-hot conditions induces elevated body temperature, cardiovascular strain and altered metabolism that can cause thermal discomfort, impaired aerobic performance and increased risk of serious heat illness (Nybo et al., 2014; Sawka et al., 2011). Heat acclimatization confers biological adjustments that reduce these negative effects of heat stress (Horowitz, 2014; Sawka et al., 1996, 2011; Taylor, 2014). Heat acclimatization, or acclimation, develops respectively through repeated natural (acclimatization) or artificial (acclimation) heat exposures that are sufficiently stressful to elevate both core and skin temperatures, that induce profuse sweating (Périard et al., 2015; Sawka et al., 2003).
INTRODUCTION OF HEAT ACCLIMATIZATION
The magnitude of biological adaptations induced by heat acclimatization depends largely on the intensity, duration, frequency and number of heat exposures (Périard et al., 2015; Sawka et al., 2003; Taylor, 2014). Even resting in the heat or exercising in a temperate environment allows for some limited acclimatization, exercise in the heat is the most effective method to develop heat acclimatization. Usually, about 7-14 d of heat exposure are needed to induce heat acclimatization. Optimal heat acclimatization requires a minimum daily heat exposure of about 90 min (can be extended to 2 h and broken into two, 1 h exposures) combined with aerobic exercise, rather than resistance training. Athletes should gradually increase the exercise intensity and duration, or just the heat exposure duration, each day of heat acclimatization.
During the initial exercise-heat exposure, physiological strain is high, as manifested by elevated core temperature and heart rate. The physiological strain induced by the same exercise-heat stress decreases each day of acclimatization. Figure 1 provides the heart rate, rectal temperature and mean skin temperature responses of persons before and during exercise each day of a 10 d dry-heat acclimatization program (Eichna et al., 1950). Through daily exercise in a hot climate, most of the improvements in heart rate, skin and core temperatures, and sweat rate are achieved during the first week of exposure (Pandolf, 1998; Sawka et al., 1996). The heart rate reduction develops most rapidly in 4-5 d, and after 7 d the heart rate reduction is essentially complete. The thermoregulatory benefits from heat acclimatization are generally complete by 10-14 d of exposure, but small additional benefits can occur afterwards (Sawka et al., 1996).
Heat acclimatization gradually disappears if not maintained by continued repeated exercise-heat exposures (Pandolf, 1998). The benefits of heat acclimatization are retained for ~1 wk and then decay with about 75% lost by ~3 wk, once heat exposure ends. During this period, re-acclimatization occurs more rapidly than the initial acclimatization when re-exposed to heat (Weller et al., 2007). A day or two of intervening cool weather will not interfere with acclimatization to hot weather. In addition, after achieving heat acclimatization, train-ing and heat acclimatization can be interspersed by every second or third day (Périard et al., 2015; Sawka et al., 2003).

Aerobically trained athletes can induce heat acclimatization more rapidly (as much as 50%) and retain its benefits longer than athletes with low aerobic fitness (Armstrong & Pandolf, 1988; Pandolf, 1998). Aerobic exercise training in temperate climates can reduce physiological strain and modestly improve exercise capabilities in the warm-hot climates (Périard et al., 2015), but such aerobic training programs alone cannot replace the benefits of heat acclimatization (Armstrong & Pandolf, 1988). Figure 2 compares the impact of an aerobic training program with heat acclimatization on reducing physiological strain and improving endurance during exercise-heat stress (Cohen & Gisolfi, 1982). After completing an initial (pre-training) exercise-heat test (4 h at ~35% maximal aerobic power in hot-dry conditions), the subjects completed the training program (1 h/day, 4 times/wk, for 11 wk in temperate conditions) and repeated the exercise-heat test. Thereafter, subjects completed a heat acclimatization program (35% maximal aerobic power, 4 h/d for 8 d) and again completed the exercise-heat test. Although aerobic training reduced physiological strain and improved endurance, these improvements were very modest compared to those obtained by heat acclimatization (Cohen & Gisolfi, 1982). Thus given that heat stress impairs aerobic performance, athletes need to integrate heat acclimatization programs while still maintaining aerobic conditioning. Although previous evidence suggests that heat acclimatization might be an effective method of augmenting the benefits of aerobic training in cool conditions (Lorenzo et al., 2010; Scoon et al., 2007); but such findings are not universal (Karlsen et al., 2015).

PHYSIOLOGICAL ADAPTATIONS
Heat acclimatization improves thermal comfort and submaximal and maximal aerobic exercise capabilities in warm-hot weather (Gonzalez & Gagge, 1976; Lorenzo et al., 2010; Nielsen et al., 1993; Racinais et al., 2015). Table 1 provides a brief description of the functional outcomes and biological adaptations associated with heat acclimatization (Sawka et al., 2011). The benefits of heat acclimatization are achieved by improved sweating and skin blood flow responses, improved cardiovascular stability (ability to sustain blood pressure and cardiac output), better fluid-electrolyte balance and a lowered metabolic rate (Périard et al., 2015; Sawka et al., 1996, 2011). Heat acclimatization is specific to the climate (desert or jungle) and physical activity level (Sawka et al., 2003). However, heat acclimatization to desert or tropical climates can markedly improve exercise capabilities in the other hot climate, but to a lesser extent as when acclimatizing in the same climate as the competition venue.
The effect of heat acclimatization on aerobic exercise performance can be quite dramatic, such that acclimatized subjects can easily complete tasks in the heat that earlier were difficult or impossible (Sawka et al., 1996, 2003). For example, the decrement in perform-ance during a self-paced time-trial in the heat is also partly recovered after 1 wk of acclimatization and almost fully restored after 2 wk of acclimatization (Racinais et al., 2015). The improved exercise capability and improved cardiovascular stability likely change in parallel. Figure 3 demonstrates improved aerobic exercise capability and cardiovascular stability with heat acclimatization. When 45 subjects attempted a 20-km march in a desert climate, 20 subjects suffered from syncope during the initial day, while by day 5 of the acclimatization program, no cases of syncope occurred (Bean & Eichna, 1943).
The three classic signs of heat acclimatization are lower heart rate, lower core temperature and higher sweat rate during exercise-heat stress (Sawka et al., 1996, 2011; Taylor, 2014). In addition, skin temperatures are often lower and sweating starts earlier and at a lower core temperature after heat acclimatization (Nadel et al., 1974). The sweat glands also become resistant to fatigue so that higher sweat rates can be sustained, particularly in high humidity climates (Gonzalez et al., 1974; Sawka et al., 1996). Earlier and greater sweating improves evaporative cooling (if the climate allows evaporation) and reduces body heat storage and skin temperature. Lower skin temperatures will decrease the cutaneous blood flow required for heat balance (because of greater core-to-skin temperature gradient) and reduce cutaneous venous compliance so that blood volume is redistributed from the peripheral to the central circulation (Sawka et al., 2011). All of these factors reduce cardiovascular strain and enhance exercise-heat performance.
Fluid balance improvements from heat acclimatization include better matching of thirst to body water needs (Bean & Eichna, 1943; Eichna et al., 1945; Périard et al., 2015), increased total body water and increased blood volume (Mack & Nadel, 1996; Sawka & Coyle, 1999). An unacclimatized person may secrete sweat with a sodium concentration of 60 mmol.L-1 or higher and, if sweating profusely, can lose large amounts of sodium (Sawka et al., 1996). With heat acclimatization, the sweat glands conserve sodium by secreting sweat with a sodium concentration as low as 10 mmol.L-1. The retention of sodium is likely an important contributor to the increased total body water (Mack & Nadel, 1996). Athletes need to ensure that they consume adequate amounts of sodium (via food and beverage), particularly early in the acclimatization process, as salt deficits can lead to dehydration despite consuming plenty of fluids (Mack & Nadel, 1996).
The repeated heating of body tissues results in Acquired Thermal Tolerance (ATT), which refers to molecular adaptations from a severe non-lethal heat exposure that allows the organism to survive a subsequent and otherwise lethal heat exposure (Horowitz, 2014). Heat acclimatization and ATT are complementary as acclimatization reduces heat strain and thermal tolerance increases survivability to a given heat load (Sawka et al., 2011).
Acquired Thermal Tolerance is associated with heat shock proteins (HSP) binding to denatured or nascent cellular polypeptides and providing protection and accelerating repair from heat stress, ischemia, monocyte toxicity and ultraviolet radiation (Horowitz, 2014; Sawka et al., 2011). These HSPs can modulate host defense, inflammatory cytokine production and protect against endotoxin exposure, which should protect against the “systemic inflammatory response syndrome” associated with exertional heat stroke (Hasday et al., 2014; Horowitz, 2014; Leon and Bouchama, 2015; Welc et al., 2013). In addition, HSP induction may be associated with improved aerobic capabilities in dogs (Bruchim et al., 2014). Heat acclimation does increase HSP levels and induction capabilities in humans (McClung et al., 2008). Both heat exposure and high-intensity aerobic exercise elicit HSP synthesis; however, the combination of aerobic exercise and heat exposure elicits a greater HSP response than either stressor does independently (Skidmore et al., 1995).
HEAT ACCLIMATIZATION STRATEGIES FOR ATHLETES
Most experimentally tested heat acclimatization strategies were developed for occupational/military settings and not for competitive athletes (Périard et al., 2015). Competitive athletes are fitter and participate in events requiring higher metabolic intensities. Therefore, the “specificity of training” and “specificity of adaptation” principles might require higher intensity exercise bouts than what has been experimentally tested. Indeed, most heat acclimatization protocols were conducted over many days eliciting a “slow” adaptation. However, athletes may rapidly travel from a temperate environment to a warm-hot climate, or from a humid heat to a dry heat climate, and may need a more rapid (and complete) induction of heat acclimatization to optimize performance.
The exercise-heat acclimatization phenotype is generally achieved through one of three induction pathways: i) constant metabolic rate; ii) self-paced and iii) controlled hyperthermia, or isothermic heat acclimation (Périard et al., 2015). The magnitude of adaptation may also relate to the induction pathway as Taylor (2014) has argued that repeated exposure to a constant metabolic rate regimen (i.e., traditional heat acclimatization) results in a less complete adaptation, whereas the progressive overload approach (e.g., controlled hyperthermia to a given core temperature) likely induces more complete heat acclimatization. It has recently been proposed that a controlled exercise intensity protocol whereby a given level of cardiovascular strain (e.g., heart rate) is maintained during daily exercise-heat exposure may further optimize adaptations (Périard et al., 2015).
To optimize performance, the exercise-heat stimulus should as closely as possible simulate the expected climate-exercise conditions during competition. However, this may require a gradual increase in the climatic heat stress, exercise intensity and duration and there may be trade-offs made by the athlete. For example, it has been shown that low-intensity long duration exercise elicits similar heat acclimatization benefits (i.e., reduced exercising heart rate, core temperature and metabolism) to that of moderate-intensity short-duration exercise (Houmard et al., 1990).
Heat acclimatization in a dry environment confers a substantial advantage in humid heat, but the physiological and biophysical differences between dry and humid heat lead one to expect that humid heat acclimation would produce somewhat different physiological adaptations from dry heat acclimation. The crossover benefits of humid and dry heat acclimatization and the benefits at higher exercise intensities have not been well studied. If heat acclimatization needs to be induced for both dry and humid heat, and if rapidity of induction is important, then we postulate that first acclimatizing athletes to dry heat (producing adaptations to sweating with some cardiovascular benefits) and secondly acclimatizing athletes to humid heat (likely inducing greater fluid regulatory and cardiovascular adaptations) might be most efficacious.
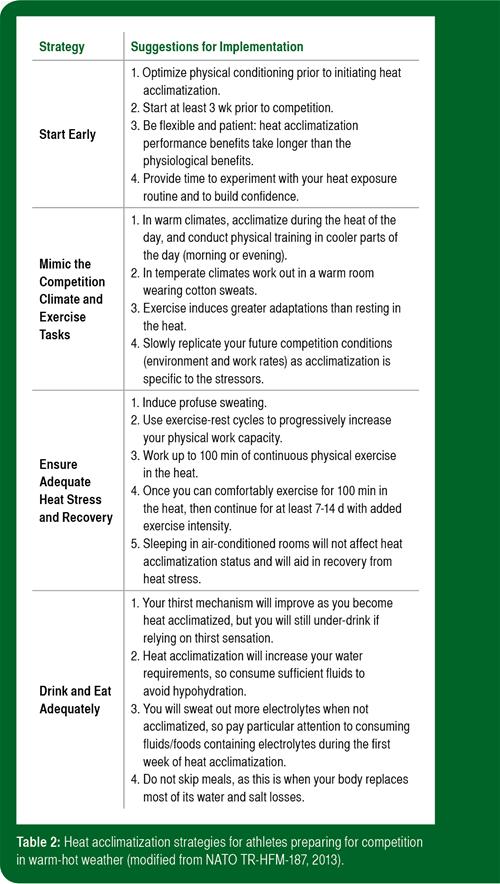
PRACTICAL IMPLICATIONS
Table 2 provides some strategies and suggestions for trainers, coaches and athletes to optimally induce heat acclimatization prior to sports competitions (Task Group HFM-187, 2013). The strategies are to start early, mimic the competition climate and exercise tasks, ensure adequate heat stress and recovery, and to eat and drink adequately.
SUMMARY
Heat acclimatization (or acclimation) is a biological adaptation that reduces physiological strain (e.g., heart rate and body temperatures), improves comfort, improves exercise capacities and reduces the risks of serious heat illness during exposure to heat stress. The biological adaptations include integrated thermoregulatory, cardiovascular, fluid-electrolyte, metabolic and molecular responses. Heat acclimatization occurs when repeated exercise-heat exposures are sufficiently stressful to invoke profuse sweating and elevate body temperatures. Generally about 1-2-wk of daily exposures of 90 min are required; but highly aerobic fit athletes can heat acclimatize in half that time. Heat acclimatization is specific to the climatic heat stress (desert or tropic) and physical exercise intensities the athletes are exposed to, which should simulate the expected competitive environment. There are strategies and suggestions that trainers, coaches and athletes can follow to optimally induce heat acclimatization prior to sports competition.
REFERENCES
Armstrong, L.E., and K.B. Pandolf (1988). Physical training, cardiorespiratory physical fitness and exercise-heat tolerance. In K.B. Pandolf, M.N. Sawka and R.R. Gonzalez (eds.) Human Performance Physiology and Environmental Medicine at Terrestrial Extremes. Benchmark Press, Indianapolis, IN, pp. 199-226.
Bean, W.B., and L.W. Eichna (1943). Performance in relation to environmental temperature: reactions of normal young men to simulated desert environment. Fed. Proc. 2:144-158.
Bruchim, Y., I. Aroch, A. Eliav, A. Abbas, I. Frank, E. Kelmer, C. Codner, G. Segev, Y. Epstein, and M. Horowitz. (2014). Two years of combined high-intensity physical training and heat acclimatization affects serum lymphocyte and serum HSP70 in purebred military working dogs. J. Appl. Physiol. 117:112-118.
Cohen J.S., and C.V. Gisolfi (1982). Effects of interval training on work-heat tolerance of young women. Med. Sci. Sports Exerc. 14:46-52.
Eichna, L.W., C.R. Park, N. Nelson, S.M. Horvath, and E.D. Palmes (1950). Thermal regulation during acclimatization in a hot, dry (desert type) environment. Am. J. Physiol. 163:585-597.
Eichna, L.W., W.B. Bean, W.F. Ashe, and N.G. Nelson (1945). Performance in relation to environmental temperature. Reactions of normal young men to hot, humid (simulated jungle) environment. Bull. Johns Hopkins Hosp. 76:25058.
Gagge A.P., and R.R. Gonzalez (1996). Mechanisms of heat exchange: biophysics and physiology. In: M.J. Fregly and C.M. Blatteis (eds.) Handbook of Physiology. Environmental Physiology. Bethesda, MD: Am. Physiol. Soc., sect. 4, pp. 45-84.
Gonzalez R.R., and A.P. Gagge (1976). Warm discomfort and associated thermoregulatory changes during dry, and humid-heat acclimatization. Israeli J. Med. Sci. 12:804-807.
Gonzalez, R.R., K.B. Pandolf and A.P. Gagge (1974). Heat acclimation and decline in sweating during humidity transients. J. Appl. Physiol. 36:419-425.
Hasday, J.D., C. Thompson, and I. Singh (2014). Fever, immunity, and molecular adaptations. Compr. Physiol. 4:109-148.
Horowitz, M. (2014). Heat acclimation, epigenetics, and cytoprotection memory. Compr. Physiol. 4:199-230.
Houmard J.A., D.L. Costill, J.A. Davis, J.B. Mitchell, D.D. Pascoe, and R. Robergs (1990). The influence of exercise intenisty on heat acclimation in trained subjects. Med. Sci. Sports Exerc. 22:615-620.
Karlsen, A., S. Racinais, M.V. Jensen, B.J. NØrgaard, T. Bonne and L. Nybo (2015). Heat acclimation does not improve VO2max or cycling performance in a cool climate in trained cyclists. Scand. J. Med. Sci. Sports. 25:269-276.
Leon, L. R. and A. Bouchama (2015). Heat stroke. Compr. Physiol. 5:611-647.
Lorenzo S., J.R. Halliwill, M.N. Sawka, and C.T. Minson (2010). Heat acclimation improves exercise performance. J. Appl. Physiol. 109:1140-1147.
Mack, G.W., and E.R. Nadel (1996). Body fluid balance during heat stress in humans. In: M.J. Fregly and C.M. Blatteis (eds.) Handbook of Physiology: Environmental Physiology, New York: Oxford University Press, Bethesda, MD: Am. Physiol. Soc., sect. 4, pp. 187-214.
McClung J.P., J.D. Hasday, J. Hr, S.J. Montain, S.N. Cheuvront, M.N. Sawka, and I. Singh (2008). Exercise-heat acclimation in humans alters baseline levels and ex vivo heat inducibility of HSP72 and HSP90 in peripheral blood mononuclear cells. Am. J. Physiol. 294:R185-R191.
McLellan, T.M., H.A.M. Daanen, and S.S. Cheung (2013). Encapsulated environment. Compr. Physiol.. 3:1363-1391.
Nadel, E.R., K.B. Pandolf, M.F. Roberts, and J.A.J. Stolwijk (1974). Mechanisms of thermal acclimation to exercise and heat. J. Appl. Physiol. 37:515-520.
Nielsen, B., J.S.R. Hales, S. Strange, N.J. Christensen, J. Warberg, and B. Saltin (1993). Human circulatory and thermoregulatory adaptations with heat acclimation and exercise in a hot, dry environment. J. Physiol. 460:467-485.
Nybo, L., P. Rasmussen, and M.N. Sawka (2014). Performance in the heat-physiological factors of importance for hyperthermia-induced fatigue. Compr. Physiol. 4:657-689.
Pandolf, K.B. (1998). Time course of heat acclimation and decay. Int. J. Sports Med. 19:S157-S160.
Périard, J.D., S. Racinais, and M.N. Sawka (2015). Adaptation and mechanisms of human heat acclimation. Scand. J. Med. Sci. Sports. 25:S20-S38.
Racinais S, J.D. Périard, A. Karlsen, and L. Nybo (2015). Effect of heat and heat-acclimatization on cycling time-trial performance and pacing. Med. Sci. Sports Exerc. 47:601-606.
Sawka, M.N., and E.F. Coyle (1999). Influence of body water and blood volume on thermoregulation and exercise performance in the heat. Exerc. Sport Sci. Rev. 27:167-218.
Sawka, M.N., C.B. Wenger, and K.B. Pandolf (1996). Thermoregulatory responses to acute exercise-heat stress and heat acclimation. In: M.J. Fregly and C.M. Blatteis (eds) Handbook of Physiology, Section 4, Environmental Physiology. Oxford University Press, New York, Section 4, pp. 157-185.
Sawka, M.N., S.N. Cheuvront, and M.A. Kolka (2003). Human adaptations to heat stress. In: H. Nose, G.W. Mack and K. Imaizumi (eds.) Exercise, Nutrition and Environmental Stress, Traverse City, MI: Cooper Publishing, 3:129-153.
Sawka, M.N., L.R. Leon, S.J. Montain, and L.A. Sonna (2011). Integrated physiological mechanisms of exercise performance, adaptation, and maladaptation to heat stress. Compr. Physiol. 1:1883-1928.
Scoon, G.S., W.G. Hopkins, S. Mayhew, and J.D. Cotter JD (2007). Effect of post-exercise sauna bathing on the endurance performance of competitive male runners. J. Sci. Med. Sport 10:259-262.
Skidmore, R., J.A. Gutierrez, V. Guerriero Jr., and K.C. Kregel (1995). HSP70 induction during exercise and heat stress in rats: Role of internal temperature. Am. J. Physiol. 268: R92-R97.
Task Group HFM-187 (2013). Management of heat and cold stress guidance to NATO medical personnel. RTO Technical Report AC/323(HFM-187)TP/496, North Atlantic Treaty Organization.