Maria T.E. Hopman, Ph.D., Rob A. Binkhorst, Ph.D.
SPORTS SCIENCE EXCHANGE
SPINAL CORD INJURY AND EXERCISE IN THE HEAT
SSE#66-Volume 10 (1997), Number 3
Maria T.E. Hopman, Ph.D.
Member, GSSI Board of Advisors for Science and Education, Europe
Department of Physiology, University of Nijmegen
Rob A. Binkhorst, Ph.D.
Department of Physiology, University of Nijmegen
The Netherlands
KEY POINTS
1. The capacity to regulate body temperature and continue exercise is a function of four factors
- the amount of heat produced in the body;
- the transport of heat from the muscles to the skin;
- the ability to dissipate heat to the environment;the capability of the body to make other physiological adjustments in order to continue regulating the temperature.
2. During physical training, the athlete who is disabled with a spinal cord injury (SCI), like the able-bodied athlete, faces the same risks of heat stress. However, the injury does affect this athlete's circulating blood volume, sweat production, and temperature regulation, and therefore can adversely influence thermoregulatory capabilities.
3. The greatest risk for heat stress appears to exist in individuals with SCI above the sixth thoracic vertebra because they are unable to increase heart rate to sustain cardiac output when blood must flow to both the muscle and the skin and because they have a reduced sweating capacity.
INTRODUCTION
Opportunities to compete in the Para-Olympics, advances in medical treatment and therapies for functional recovery of the disabled, and the recognition that physical activity is beneficial for the health of everyone, abled or disabled, have contributed to increased participation of disabled individuals in regular physical exercise. Like able-bodied athletes, disabled athletes face limitations to performance-fatigue, nutrition and fluid needs, and the possibility of heat exhaustion. In this report, we will discuss thermoregulation and cardiovascular function in the disabled athlete. We will make several recommendations to reduce the chance of heat illness and to delay fatigue in the disabled athlete.
PRINCIPLES OF THERMOREGULATION
At rest, the body produces heat at a rate of approximately 70 W, or 1 kcal/min, whereas the rate may be 2100 W (30 kcal/min) during maximal exercise in well-trained athletes. The latter rate, however, is dependent on the type of work and the mechanical efficiency for performing external work. The energy that is not dissipated as heat from the body will be transformed into mechanical work. The most efficient type of human exercise is bicycling, with an efficiency of about 20-23%. The remaining energy, about 80% of the metabolic energy, will be lost as heat in the body. Technically, at rest and during activities such as walking and running, i.e., activities without any external resistance, little or no external work is performed so almost all of the metabolic energy will be dissipated by the body as heat.
The total amount of heat released by the body is not only dependent on the rate of metabolism and the mechanical efficiency but also on the duration of the activity. For example, the total amount of heat liberated by the body during 8 h of sleep is 115 kJ (480 kcal), whereas the total amount will not exceed 35 kJ (150 kcal) for the athlete performing exercise at a level of 2100 W (30 kcal/min). The reason is that the athlete will only be able to perform at this level for about 5 min.
Still, an athlete produces a large amount of heat as a consequence of prolonged high-intensity exercise. The heat will accumulate in the body if the avenues of heat dissipation-evaporation of sweat plus radiation and convection of heat from the skin-have an insufficient capacity or are not available. The body temperature will then increase to a very high value. If a person ran at a speed of 15 km/h (10 mi/h) and the body could not release the heat for some reason, the internal temperature would increase above 40° C (104 within 15 min. Consequently, prolonged heavy exercise in the heat could lead to life threatening heat stroke, the most extreme type of possible heat illnesses.
During exercise, body heat is generated primarily in the active muscles. Transport mechanisms, which include the circulating blood and conduction between body tissues, bring the heat to the skin. At the skin, evaporation, convection, radiation, and, far less important, conduction, can transfer the heat from the skin to the environment. Certain situations can impede heat release. For example, the evaporation of sweat will stagnate when the humidity of the air is high. Also, when the air temperature is higher than the skin temperature, convection, radiation and conduction will result in the transfer of heat from the air to the body.
To maintain a body temperature that is within a safe range, the following factors must be considered:
- a. the intensity and the duration of the exercise and the body's mechanical efficiency for the effort being performed (this establishes the amount of heat released by the body);
- b. the blood circulation and blood volume (these determine the transport of heat from the muscles to the skin);
- c. the amount of sweat produced and the temperature and humidity of the environment (these determine how much heat can be given off to the environment);
- d. the capability of the body to make other physiological adjustments in order to continue regulating the temperature. For instance, as body heat continues to be generated with exercise over time, the blood flow is diverted away from the liver and other internal organs to the active muscles and the skin. This redistribution of blood flow enhances muscle function and heat dissipation without decreasing cardiac output or causing great disturbances in blood pressure.
The reader is referred to more detailed information on this topic in an earlier issue of Sports Science Exchange written by Nadel (1990).
CHARACTERISTICS OF INDIVIDUALS WITH A SPINAL CORD INJURY
In athletes who have spinal cord injuries (SCI), three of the four factors necessary for regulating temperature are affected (Binkhorst, 1995; Hopman, 1993, 1994). Specifically, circulating blood volume, sweat production, and skin surface area for effective heat transfer to the environment are affected and can impair the athlete's ability to stay cool during sustained exercise training. In this section, we'll discuss how these factors are affected and how they might alter thermoregulation in the disabled athlete.
The extent to which the circulation is affected depends on the level and severity (incomplete or complete) of the spinal cord lesion. Figure 1 identifies the levels of possible injury. After a complete lesion above the 6th thoracic vertebra (T6) the sympathetic regulation of the heart will be affected; hence, heart rate remains low, and the myocardial contractile force is impaired. The distribution of blood below the lesion is impaired due to a lack of vasoconstriction in the internal organs of the abdomen and the pelvis; this diminishes the ability to redistribute blood during exercise. In addition, blood flow in muscles and skin as well as the sweat gland activity in the affected skin below the lesion are impaired.
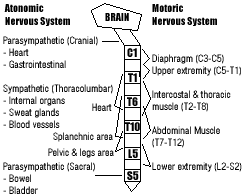 | Figure 1: Diagram of the central nervous system and the neuronal outflows for the motoric nervous system (skeletal muscle innervation) and autonomic nervous system (internal organs, blood vessels, sweat glands). General innervations related to the level of the spinal cord are indicated. Based on this information spinal cord-injured individuals can be classified into groups. |
A complete lesion between T6 and T10 will not affect cardiac function. However, sympathetic vasoconstriction in the abdominal and pelvic organs is absent below such a lesion. The regulation of the sweat glands and blood flow to the muscles and skin below the lesion will also be impaired.
With a complete lesion at or below T10, there will be a loss of the central regulation of vasoconstriction in the pelvic area, a diminished blood flow in the legs (muscles and skin), and a reduced activity of the sweat glands below the lesion.
It may thus be expected that the physiological responses of individuals with SCI during exercise in the heat are different from able-bodied individuals and that the changes will depend on the level and completeness of the lesion.
INDIVIDUALS WITH SCI WHO EXERCISE IN THE HEAT
Typically, individuals with SCI perform exercise by using their arms to propel the wheelchair in which they sit. The 8-15% mechanical efficiency of arm exercise is considerably lower than that of bicycling. Consequently, a substantial part of the metabolic energy in these persons will be transformed into heat in the muscles. Additionally, the thermal stress may be greater compared to leg exercise at a given metabolic rate due to cardiovascular disadvantages in supporting thermoregulation (diminished venous return, lower stroke volume) (Sawka, 1986).
A series of experiments reported by Hopman (1994) demonstrated the effect of prolonged exercise in the heat on individuals with SCI. Three groups of trained paraplegic men (P) with complete lesions and a control group (C) participated in these experiments. The levels of the lesions, which had existed for at least 2 y, were T2-T6 (P1), T7-T9 (P2) and T10-T12 (P3). The subjects performed 45 min of arm-cranking exercise in a wheelchair at an intensity of 40% of their individual maximal loads, i.e., 462 W (6.6 kcal/min), 362 W (5.2 kcal/min), 333 W (4.8 kcal/min), and 282 W (4.0 kcal/min), respectively, for the groups C, P3, P2, and P1. Because of the different power outputs, the amounts of heat to be dissipated differed among groups even though consistent environmental conditions were maintained (35° C air temperature, 70% relative humidity, and a wind velocity below 0.1 m/s). The subjects drank water ad lib. Metabolic rate, cardiac output, heart rate, rectal temperature, skin temperature at sites above and below the lesions, and sweat loss and fluid intake were assessed.
BLOOD CIRCULATION IN SCI
One finding in the Hopman (1994) study was that the individuals with a spinal cord lesion above T6 were at risk for adverse consequence of heat stress during exercise. During exercise in the heat the metabolic rate of each group remained fairly stable over the 45 min, suggesting that mechanical efficiency remained consistent and that the subjects did not become exhausted. However, those with SCI above T6 experienced a decrease in cardiac output during the exercise due to a decrease in stroke volume coupled with a relatively small rise in heart rate. The latter is a consequence of the lack of sympathetic innervation of the heart as a result of the lesion. The average heart rate in individuals with SCI above T6 reached only 110 beats/min, whereas the other groups had values of 130-150 beats/min at the end of exercise. Attaining higher rates in the able bodied subjects and those with SCI below T6 contributed to a constant cardiac output even though the stroke volume decreased during exercise as the central blood flow was reduced as a consequence of increasing the skin blood flow for thermoregulation (Gass & Camp, 1987).
What are the implications of this for patients and athletes with SCI above T6? During prolonged work in the heat, the circulation must maintain an adequate blood pressure to supply the active muscles with blood and simultaneously shift blood to the skin and away from the liver, kidneys, and gut. If the cardiac output cannot meet both of these demands, the first priority will be to maintain the blood pressure (Binkhorst & Hopman, 1995). The skin will consequently receive less blood, the body temperature will rise, and the risk of heat stroke increases. A decrease in cardiac output such as that occurring in those with SCI above T6 indicates that the circulatory system cannot meet the increased circulatory requirements of exercising in the heat.
BODY TEMPERATURES IN SCI
At rest and at the start of exercise the temperatures of the skin (Ts) covering the torsos and legs of the able bodied subjects in the Hopman (1994) study were similar (35° C), indicating an even distribution of blood flow to the skin in all regions of the body. In contrast, in all subjects with SCI, temperature of the legs (32° C ) was about 4° C lower than that of the upper body (near 36° C), indicating an impaired skin blood flow below the lesion. At the end of the exercise period, the torso and leg skin temperatures (36-37° C) were similar for both the able bodied subjects and those with SCI below T6, whereas for subjects with SCI above T6, the Ts of the torso (38° C) was clearly warmer than that of the legs (34° C). Some of the heat produced during exercise is conveyed by the blood to the legs of the SCI subjects, which explains the increase of Ts below the lesion. In P1 the moderate increase in Ts of the legs (compared to the other P-groups) may be due to the lower metabolic rate achieved during exercise and to a more severe disturbance in blood distribution. The higher Ts of the torsos of those with SCI above T6 compared to individuals with lower lesions and the able bodied may be related to an impairment in sweat production in the P1 subjects.
The exercise and environment used in the Hopman (1994) experiments does not lead to extreme body temperatures in control or SCI-individuals. However, based on the trends in rectal temperature (Gass et al., 1988) and total body temperature (Tb= 0.2 Ts + 0.8 Tr), longer lasting exercise in those with SCI above T6 may produce body temperatures that put these individuals at risk. Rectal temperatures (Tr) of the able bodied subjects and all SCI subjects (initially 36.1-37.4° C) increased by 0.5-0.8° C. Body heat content increased by 2-3% for C and P. The highest Tr was 38.0° C in the able bodied and in those with SCI above T6. Total body temperature of the able bodied subjects and those with lesions below T6 increased from rest (35.5-36.8° C) to the end of the exercise (36.5-37.5° C) and showed a leveling off towards the end of the test. However, in subjects with SCI above T6, Tb increased almost linearly until the end of exercise (from 36.5 to 38.0° C), without showing a plateau.
SWEAT EVAPORATION IN SCI
The relatively hot environment used in the experiments of Hopman (1994) meant that the major avenue for heat dissipation was the evaporation of sweat. There was a linear relationship between the sweat produced and the heat liberated by the subjects in the four groups: 718, 425, 335, and 172 g of sweat for the able bodied and for those with SCI between T10-T12, T7-T9, and T2-T6, respectively. A linear relationship was also found between the sweat produced and the skin surface area above the lesion for active sweat regulation. Nevertheless, the fact that Tr and Ts began to level off near the end of the test indicates that sweat evaporation maintained a stable heat balance in the able bodied subjects and in those with SCI lesions below T6. In contrast, subjects with SCI above T6 did not reach a balance between heat production and heat dissipation during the exercise.
SUMMARY
Although heat stress during exercise is a concern for all athletes, in most cases, athletes with SCI are physiologically capable of performing arm cranking exercise in experimental studies. However, during exercise the stroke volumes of persons with SCI tend to be lower than those of able-bodied individuals (Hopman et al., 1992; Davis et al., 1990). The lower stroke volume in SCI may be a limiting factor in exercise performance, especially in the heat when stroke volume decreases and heart rate attempts to compensate to maintain a stable cardiac output (cardiac drift).
For SCI individuals with lesions above T6, the combination of exercise and heat may lead to circulatory impairments and/or to unacceptably high body temperatures. In these individuals, the heart rate plateaus at a lower value than that in able-bodied individuals or individuals with a SCI lesions at lower sites. Cardiac output can no longer maintain blood flow to the muscle and the skin in SCI individuals with lesions above T6. Consequently, skin blood flow is diminished, and body temperature increases undesirably .
In more extreme climatic situations (high temperature and high humidity) and/or during high-intensity exercise, athletes with SCI may be at greater risk of heat illness than are able-bodied athletes. Furthermore, the effects of heat stress can be exaggerated when the individual is not fully hydrated. It is critical, therefore, for SCI athletes, their coaches, and their athletic trainers, to be conscious of the environmental conditions; to make appropriate changes in training intensity, environment (indoors or outdoors in the heat), and duration, and to ensure proper hydration during exercise. As with able-bodied athletes, SCI athletes should wear light clothing during exercise in the heat, should be aware of warning signs of potential heat illness, e.g., fatigue, headache, dizziness; and should become gradually acclimatized to exercise in hot environments.
References
Binkhorst, R.A., and M.T.E. Hopman (1995). Heat balance in paraplegic individuals during arm exercise at 10 and 35° C. Med. Sci. Sports Exerc. 27: s83 (abstract).
Davis, G.M., F.J. Servedio, R.M. Glaser, and A.G. Suryaprasad (1990). Cardiovascular responses to arm-cranking and FNS-induced leg exercise in paraplegics. J. Appl. Physiol. 69:671-677.
Gass, G.C., and E.M. Camp (1987). Effects of prolonged exercise in highly trained traumatic paraplegic men. J. Appl. Physiol. 63:1846-1852.
Gass, G.C., E.M. Camp, E.R. Nadel, T.H. Gwinn, and P. Engel (1988). Rectal and rectal vs. esophageal temperatures in paraplegic men during prolonged exercise. J. Appl. Physiol. 64:2265-2271.
Hopman, M.T.E. (1994). Circulatory responses during arm exercise in individuals with paraplegia. Int. J. Sports Med. 15: 126-131.
Hopman, M.T.E., B. Oeseburg, and R.A. Binkhorst (1992). Cardiovascular responses in paraplegic subjects during arm exercise. Eur. J. Appl. Physiol. 65:73-78.
Hopman, M.T.E., B. Oeseburg, and R. Binkhorst. (1993). Cardiovascular responses in paraplegics to prolonged arm exercise and thermal stress. Med. Sci. Sports Exerc. 25: 577-583.
Nadel, E.R. (1990). Limits imposed on exercise in a hot environment. Sports Sci. Exchange 3 (27).
Sawka, M.N. (1986). Physiology of upper-body exercise. In: K.B. Pandolf (ed.) Exercise and Sport Sciences Reviews, vol. 14. New York: Macmillan, pp. 175-211.
Sawka, M.N., R.R. Gonzalez, L.L. Drolet, and K.B. Pandolf (1984). Heat exchange during upper- and lower-body exercise. J. Appl. Physiol. 57: 1050-1054.