Graeme L. Close
KEY POINTS
- Vitamin D is associated with numerous important biological actions relevant to the athlete including regulating bone health, immune function, cell cycle and skeletal muscle homeostasis.
- Vitamin D deficiency has been linked to a number of adverse health outcomes.
- Athletic populations show markedly poor vitamin D concentrations, particularly during the winter months.
- Vitamin D deficiency is reversible through oral vitamin D supplementation and safe sun exposure.
- Well-designed randomized controlled trials are now required to establish the extent to which vitamin D may impact athletic performance.
RESEARCH REVIEW
Background
The past decade has seen a renaissance in vitamin D research. A simple PubMed search for “vitamin D” provides over 3,500 papers from 2013 compared with just over 1,000 published in 1993. This is in part due to the re-emergence of the preventable bone disorder, rickets, stimulating interest in the field. In search of a better understanding of vitamin D metabolism and function, a multiplicity of biological roles for the steroid hormone has emerged. Since the identification of the vitamin D receptor in various tissues through which vitamin D exerts many of its effects (Demay, 2006), and the generation of the vitamin D receptor knockout mouse (Li et al., 1997), there has been a vast advancement in our knowledge of the actions of vitamin D. It is now understood that aspects of innate and acquired immunity, cardiovascular health and biological processes within skeletal muscle are regulated by vitamin D.
Despite this growing understanding of the importance of vitamin D, large population-based studies provide evidence that vitamin D deficiency is common worldwide owing to a sun-shy lifestyle and poor dietary sources of vitamin D. This poses a unique problem for athletic populations as deficiency may go unnoticed but contribute to sub-optimal immune function, poor bone health and potentially perturbed muscle function and regenerative capacity. This short review will explore key considerations when assessing and interpreting the measurement of vitamin D status and protocols for supplementation with vitamin D.
What to Measure?
There are two natural forms of vitamin D, these being ergocalciferol (vitamin D2) and cholecalciferol (vitamin D3). Vitamin D2 is available in limited amounts from plant sources and in some supplements, whereas vitamin D3 is found in dietary sources such as fatty fish and dairy produce. However, the major source of vitamin D3, contributing 90% of vitamin D intake, is obtained via a photosynthetic reaction in the dermis of the skin that occurs when sufficient exposure to ultraviolet B (UVB) radiation is achieved. This exposure is dependent on clothing, ethnicity, lifestyle and importantly solar zenith, which is influenced by geographic location and time of day (Chen et al., 2007). Regardless of the route of entry, 99% of vitamin D travels bound to the vitamin D binding protein (DBP) while the remainder is bound to albumin (Chun et al., 2014). Both vitamin D2 and D3 undergo hydroxylation in the liver catalysed by 25-hydroxylase to form 25-hydroxyvitamin D (25(OH)D) and further hydroxylation in the kidneys or target tissues expressing the enzyme 1-α-hydroxylase to form the biologically active form of vitamin D, 1-α-dihydroxyvitamin2D3 (1-α,25(OH)D) (Figure 1).
When assessing vitamin D status, however, it is the product of the first hydroxylation step, 25(OH)D that is the measurement of choice. This begs the question “why is it preferable to measure a biologically inactive form of vitamin D and not the active form?” The answer is that hepatic 25-hydroxylase is regulated only by 25(OH)D concentration and no other stimuli such as parathyroid hormone (PTH), which stimulates renal production of 1α,25(OH)D when 25(OH)D concentrations are low (Kumar & Thompson, 2011). Measuring 1α,25(OH)D may therefore lead to erroneous classification of an individual’s vitamin D status, ultimately resulting in misdiagnosis. Furthermore, 1α,25(OH)D circulates in concentrations about 1,000 fold less than 25(OH)D (Broadus et al., 1980) and has a half-life of ~15 h whereas 25(OH)D has a half-life of ~15 d (Jones, 2008).
How to Measure It?
In brief, blood is typically collected via venipuncture and more recently “blood spots” from a simple finger prick from the individual being assessed. The serum (from venipuncture) or blood (from blood spot) is isolated and used in the assay being employed. There are then a number of methods to assess 25(OH)D to determine vitamin D status. Commercially available assays include:
- High pressure liquid chromatography mass spectrometry (LC-MS/MS)
- Radioimmunoassay (RIA)
- Enzyme immunoassay (EIA)
- Competitive protein binding assay (CPBA)
- Automated chemiluminescent protein binding assay (CLPBA)
- Chemiluminescent immunoassay (CLIA)
When choosing an assay, it is essential to consider the validity and reliability of the measurement tool. Notably, reports suggest that there is considerable inter-assay variability. However, it is accepted that LC-MS/MS is the most valid and reliable method for the measurement of 25(OH)D, while CLIA performs worst (Snellman et al., 2010). The Vitamin D External Quality Assessment Scheme (DEQAS) exists to ensure the analytical reliability of 25(OH)D assays. DEQAS can independently test the performance of the analytical method a laboratory implements to measure 25(OH)D and provide certification of proficiency.
What Do the Numbers Tell Us?
First, it is important to note that dependent on location, circulating concentrations of vitamin D metabolites may be expressed in different units. The two most commonly reported units of measurement are ng/mL and nmol/L, where 1 ng/mL = 2.496 nmol/L. The SI unit for vitamin D is nmol/L and will be used herein.
Following laboratory analysis via a vitamin D assay (for example LC-MS/MS), a quantitative reading is produced giving an indication of circulating vitamin D of the individual. Vitamin D2 (25(OH)D2) and D3 (25(OH)D3) may both be analysed and referred together as total serum 25(OH)D. At present, there is disparity as to what serum 25(OH)D concentrations constitute deficiency, adequacy and “optimal” status. The United States Institute of Medicine (U.S. IOM) at present provides guidelines that should be accepted until revised (Table 1).
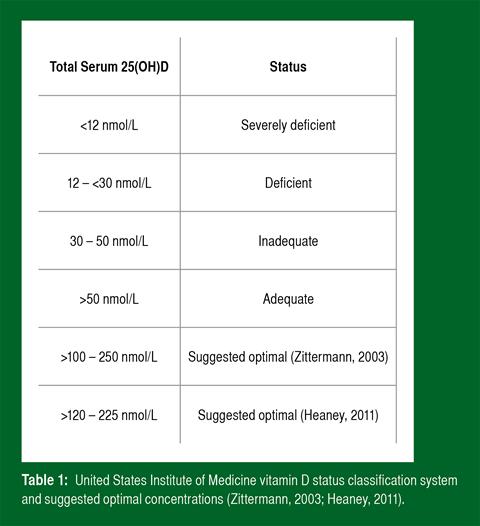
However, it is noteworthy that numerous scientific researchers disagree with the guidelines and propose that they are too conservative and outdated. From available literature and our own findings, it is evident that there is great inter-individual variation in basal vitamin D concentrations in participants tested at the same point of the year. As an example, Figure 2 demonstrates cumulative findings from a trial conducted in our laboratory during the winter months across a range of athletic disciplines. A notable observation from the data below is that the vast majority of tested athletes present with vitamin D status that is considered deficient or inadequate as suggested by the U.S. IOM.

How Do You Improve Vitamin D Status?
Before discussion of mechanisms to improve vitamin D status, there are a number of considerations that must be taken into account. As with the measurement units used for circulating metabolites of vitamin D, there is more than one unit of measurement for supplemental vitamin D. It is extremely important to distinguish between international units (IU) and μg. One hundred IU = 2.5 μg vitamin D2/D3. Clearly, confusing these two could result in extremely large or very low and ineffective doses of vitamin D.
A further consideration before supplementation is whether vitamin D2 or D3 is more effective. Quite simply, vitamin D3 is approximately 87% more potent in raising and maintaining serum 25(OH)D concentrations and produces 2- to 3-fold greater storage of vitamin D than does an equimolar amount of D2 (Heaney, 2011).
This is intuitive given the primary route of obtaining vitamin D is via dermal synthesis, a process that produces vitamin D3.
Basal vitamin D concentrations differ greatly among individuals (Figure 3) and this can have a profound impact upon the response to supplementation. Figure 3 illustrates a simple linear regression redrawn from (Close et al., 2013a) and Owens et al. (unpublished observation). The plot describes that baseline vitamin D concentration is a strong predictor of the response to supplementation i.e., those with low basal serum 25(OH)D show the greatest response to supplementation. However, individuals with similar baseline 25(OH) D may not respond to supplementation in a comparable way as genetic variation in the vitamin D binding protein gene appears to influence responsiveness to supplementation (Nimitphong et al., 2013).
With regards to dosing, serum 25(OH)D responds to supplementation in a dose-dependent manner to vitamin D3 (Heaney et al., 2003). The more appropriate question however, is what serum 25(OH)D concentration we are aiming to achieve, as this (as well as basal 25(OH)D) will indeed impact upon the dose administered. This remains a topic of hot debate and a universal serum concentration for optimization of all physiological functions affected by vitamin D is far from being established. However, it is becoming more commonly accepted that health is better with serum 25(OH)D concentrations >75 nmol/L (Heaney, 2013). To achieve serum concentrations >75 nmol/L, we have previously demonstrated that supplementation with oral vitamin D3 at a dose of 5,000 IU/d for 8 wk can effectively achieve this (Close et al., 2013a). Other authors have suggested that estimated serum concentrations needed for other health benefits are above 100 nmol/L and that a daily requirement of 9,600 IU/d is needed to maintain such serum concentrations (Garland et al., 2011). In support, Michael Holick and colleagues have demonstrated that adults in a bathing suit exposed to 1 minimal erythemal dose (MED) of tanning bed (UVB) radiation raises blood levels of vitamin D to levels equivalent to those achieved by ingesting between 10,000 and 25,000 IU of vitamin D (Holick, 2002).
Notably, these supplemental doses would be considered ~8x and ~16x of the recommended daily intake (RDI), respectively, as U.S. IOM has set the RDI for vitamin D at 600 IU/d (for young adults) and the tolerable upper intake at 4,000 IU/d. However, the U.S. IOM has also set the “no observed adverse effect limit” (NOAEL) at 10,000 IU/day. It is important to consider however, that dermal synthesis of vitamin D via UVB exposure is self-regulated with pre vitamin D being converted to inactive photoproducts when synthesis is no longer required (Holick et al., 1981). Such a biological mechanism is not available for oral ingestion; therefore, if extremely high doses are ingested above the NOAEL this may cause vitamin D toxicity, although reports of this are rare.
PRACTICAL APPLICATIONS FOR THE ATHLETE
Given the present information, there are a number of considerations when exploring practical applications of our current understanding of vitamin D status and supplementation.
- Baseline concentration of an athlete’s circulating total 25(OH) D should be measured given the inter-individual differences consistently observed.
- Measurement should be made using the most valid technique available, preferentially LC-MS/MS. The recent development of the blood spot technique may be a more practical way to obtain blood samples for athletic teams.
- According to the individual’s baseline concentration an appropriate supplementation method should be adopted. If the athlete presents with serum concentrations <75 nmol/L, then an oral 5,000 IU/d is an effective protocol to increase concentrations >75 nmol/L. If the athlete presents with severe vitamin D deficiency (<30 nmol/L) and particularly severe deficiency (<12.5 nmol/L), 10,000 IU/d may be effective to increase concentrations rapidly within 4 weeks, which may then be sustained with 5,000 IU/d.
- Regular blood sampling may be appropriate particularly during the winter months to monitor the efficacy of the supplementation protocol.
- Safe whole body minimal erythemal sun exposure bouts lasting 30 min during the summer may help to elevate total 25(OH)D without supplementation. At northerly latitudes above 50° N, this still may be difficult to achieve.
SUMMARY
In summary, data suggests vitamin D deficiency is endemic and its frequency is on the rise, a consistent observation in athletic sub-groups. This may primarily be owing to a sun-shy lifestyle and poor dietary sources of vitamin D. The cost of vitamin D deficiency is sub-optimal biological function in many tissues and therefore measurement is necessary in athletes, particularly during the winter months when sun exposure is low. Appropriate biochemical methods for vitamin D assessment should be adopted and correct interpretation of results implemented. The current RDI for vitamin D (600 IU/d) is unlikely to be efficacious to prevent deficiency in the absence of sun exposure. If necessary (upon presentation of serum 25(OH)D <75 nmol/L) it is advisable to supplement with oral vitamin D3 with doses reflecting the necessity of change required in serum 25(OH)D.
REFERENCES
Broadus, A.E., R.L. Horst, R. Lang, ET. Littledike, and H. Rasmussen (1980). The importance of circulating 1,25-dihydroxyvitamin D in the pathogenesis of hypercalciuria and renal-stone formation in primary hyperparathyroidism. N. Engl. J. Med. 302:421-426.
Chen, T.C., F. Chimeh, Z. Lu, J. Mathieu, K.S. Person, A. Zhang, N. Kohn, S. Martinello, R. Berkowitz, and M.F. Holick (2007). Factors that influence the cutaneous synthesis and dietary sources of vitamin D. Arch. Biochem. Biophys. 460:213-217.
Chun, R.F., B.E. Peercy, E.S. Orwoll, C.M. Nielson, J.S. Adams, and M. Hewison (2014). Vitamin D and DBP: The free hormone hypothesis revisited. J. Steroid Biochem. Mol. Biol. 144:132-137.
Close, G.L., J. Leckey, M. Patterson, W. Bradley, D.J. Owens, W.D. Fraser, and J.P. Morton (2013a). The effects of vitamin D3 supplementation on serum total 25(OH) D concentration and physical performance: a randomised dose-response study. Br. J. Sports Med. 47:692-696.
Close, G.L., J. Russell, J.N. Cobley, D.J. Owens, G. Wilson, W. Gregson, W.D. Fraser, and J.P. Morton (2013b). Assessment of vitamin D concentration in non-supplemented professional athletes and healthy adults during the winter months in the UK: implications for skeletal muscle function. J. Sports Sci. 31:344-353.
Demay, M.B. (2006). Mechanism of vitamin D receptor action. Ann. N. Y. Acad. Sci. 1068:204-213.
Garland, C.F., C.B. French, L.L. Baggerly, and R.P. Heaney (2011) Vitamin D supplement doses and serum 25-hydroxyvitamin D in the range associated with cancer prevention. Anticancer Res. 31:607-611.
Heaney, R.P. (2011). Assessing vitamin D status. Curr. Opin. Clin. Nutr. Metab. Care 14:440-444.
Heaney, R.P. (2013). Health is better at serum 25(OH)D above 30ng/mL. J. Steroid Biochem. Mol. Biol. 136:224-228.
Heaney, R.P., K.M. Davies, T.C. Chen, M.F. Holick, and M.J. Barger-Lux (2003). Human serum 25-hydroxycholecalciferol response to extended oral dosing with cholecalciferol. Am. J. Clin. Nutr. 77:204-210.
Heaney, R.P., P.R. Recker, J. Grote, R.L. Horst, and L.A. Armas (2011). Vitamin D3 is more potent than vitamin D2 in humans. J. Clin. Endocrinol. Metab. 96:E447-452.
Holick, M.F. (2002) Sunlight and vitamin D: both good for cardiovascular health. J. Gen. Intern. Med. 17:733-735.
Holick, M.F., J.A. MacLaughlin, and S.H. Doppelt (1981). Regulation of cutaneous previtamin D3 photosynthesis in man: skin pigment is not an essential regulator. Science 211:590-593.
Jones, G. (2008) Pharmacokinetics of vitamin D toxicity. Am. J. Clin. Nutr. 88:582S-586S.
Kumar, R., and J.R. Thompson (2011). The regulation of parathyroid hormone secretion and synthesis. J. Am. Soc. Nephrol. 22:216-224.
Li, Y.C., A.E. Pirro, M. Amling, G. Delling, R. Baron, R. Bronson, and M.B. Demay (1997). Targeted ablation of the vitamin D receptor: an animal model of vitamin D-dependent rickets type II with alopecia. Proc. Natl. Acad. Sci. USA 94:9831-9835.
Nimitphong, H., S. Saetung, S. Chanprasertyotin, L.O. Chailurkit, and B. Ongphiphadhanakul (2013). Changes in circulating 25-hydroxyvitamin D according to vitamin D binding protein genotypes after vitamin D3 or D2 supplementation. Nutr. J. 12:39.
Owens, D.J., and G.L. Close (2013). Vitamin D and athletic performance. Agro FOOD Industry Hi Tech, vol 24.
Snellman, G., H. Melhus, R. Gedeborg, L. Byberg, L. Berglund, L. Wernroth, and K. Michaelsson (2010), Determining vitamin D status: a comparison between commercially available assays. PLoS One 5:e11555.
Zittermann, A. (2003). Vitamin D in preventive medicine: are we ignoring the evidence? Br. J. Nutr. 89:552-572.